The adoption of renewable energy is exploding. Solar has been the fastest growing source of electricity for the past 19 years. It is paving the way for a future without fossil fuels. Yet, renewables face a unique challenge: they are intermittent by nature, necessitating a mechanism to ensure a steady supply of energy when the sun sets and the wind stills. This is where energy storage comes into play β it is not an exaggeration to say that batteries are one of the most important technologies we need for a successful energy transition.1
In the energy storage market, lithium-ion is currently the leading, most mature technology. While alternative chemistries like redox flow and iron air promise longer durations, they remain in pre-commercialisation stages. Sodium-ion batteries, which function similarly to lithium-ion batteries but utilise more abundant materials, could play a significant role in further reducing the cost of energy storage. China recently announced the first operational large-scale sodium-ion battery storage station, signalling the technologyβs entry into the market.
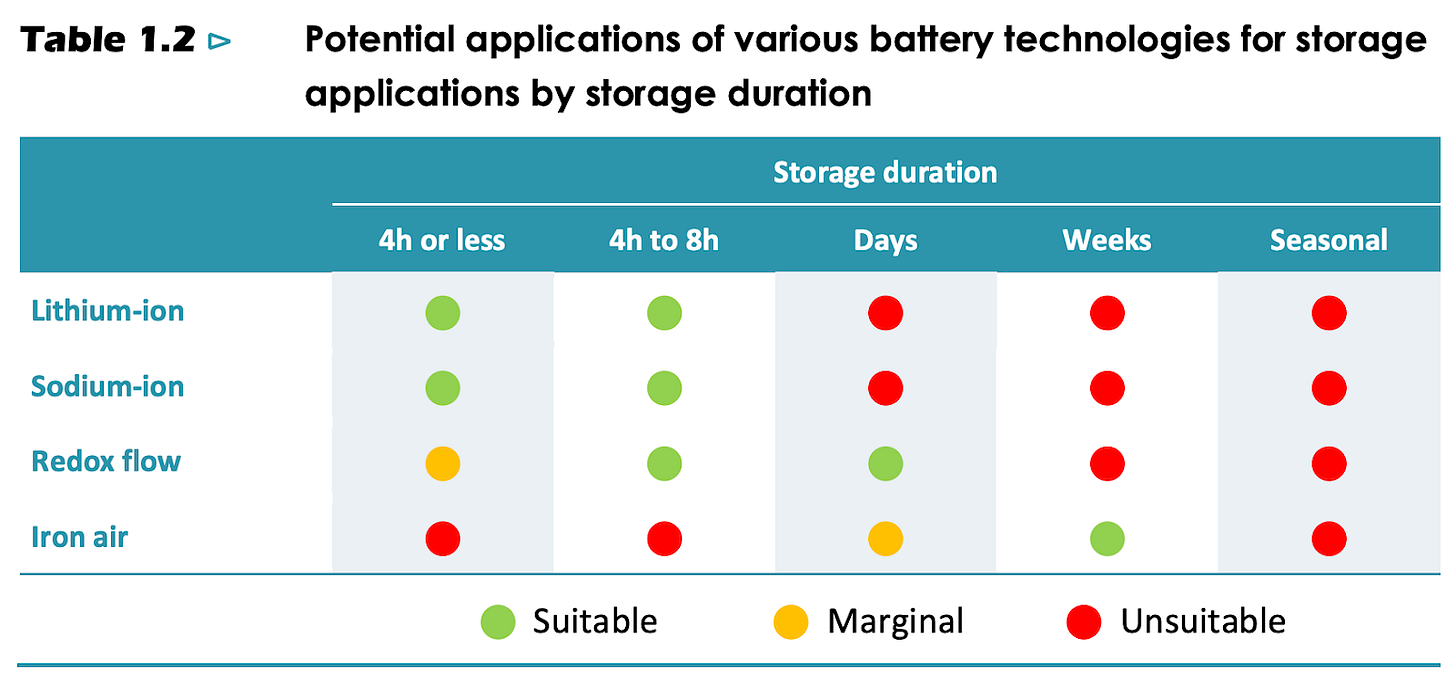
This Chartpack focuses on lithium-ion batteries, as they have reached the necessary maturity to serve short-term storage needs. 2
Part 1 examines the current state of lithium-ion technology, its manufacturing processes and the institutional bottlenecks that hinder its potential.
Part 2 will explore solutions to these bottlenecks and how overcoming them could lead to more decentralised energy systems and radically different economic outcomes.
So, letβs start from the beginningβ¦
Where are we now with batteries?
Lithium-ion batteries, like solar power and, to a lesser extent, wind, have experienced dramatic cost declines over the last few years. Unlike fossil fuels, which are finite resources, battery technology benefits from learning rates as experience is gained from their manufacture. With a historical learning rate of ~20%, the cost of battery storage is expected to continue its downward trajectory, further accelerating adoption.3
Solar when combined with batteries is becoming cost-competitive with natural gas in the US. Yet, battery storage capacity is not keeping pace with renewable energy generation. To achieve the COP28 goal of tripling renewable energy capacity by 2030, battery storage must increase fourteenfold to ensure a stable supply.
This lag may raise concerns, but the 8 TWh of planned battery manufacturing capacity globally is set to surpass the 6 TWh needed by 2030.4
Another oft-raised concern is the material requirements of batteries. The mineral requirements, while significant, donβt necessarily impede the clean energy transition. As Azeem pointed out in 2022, weβve overcome similar challenges before, such as scaling up agriculture and the oil industry despite limited technology and resources. The mineral supply problem is a patchwork of complex opportunities, not a singular, insurmountable obstacle. Technological choices, economic incentives and political pressure can adjust market development, as seen in the rapid shift away from cobalt in battery chemistries. Unlike fossil fuels, minerals like lithium and cobalt are used, reused and eventually recovered, creating recycling opportunities. In fact, 95% of batteries can now be recycled, although the industry is still nascent.
Different types of lithium-ion batteries have even cut the need for critical minerals. The dominant battery in China, LFP (lithium iron phosphate), has seen remarkable growth over the last three years. It accounts for 80% of new battery storage in 2023 and uses no problematic minerals like nickel or cobalt, but comes at the cost of lower energy density.5 Growing demand will likely spur further innovation in developing alternative battery architectures.
At Exponential View, we see both the manufacturing cost economics and technological progression of batteries as being in good stead. Advances in production processes, raw material sourcing and economies of scale have led to significant reductions in battery manufacturing costs. Simultaneously, ongoing research and development have resulted in improvements in energy density, charging speeds and battery lifespans.
But itβs still not easy
What often changes last, and is crucial for widespread adoption, are the institutional factors that determine how a technology is adopted, regulated, and integrated into society.Β
Currently, there are three major forms of institutional inertia affecting the adoption of energy storage: